Figure 9. Ensemble-averaged MSD vs. lag time and measured viscosity for 5% dextran-water solution. The data of three
independent experiments agree with each other after deterministic motion cancellation (DMC). There is a close agreement between the high-shear-rate apparent
viscosity of 5% dextran-water solution, measured with Advanced Rheometer 2000 (TA Instruments), and the value of viscosity measured with the MPTM with DMC.
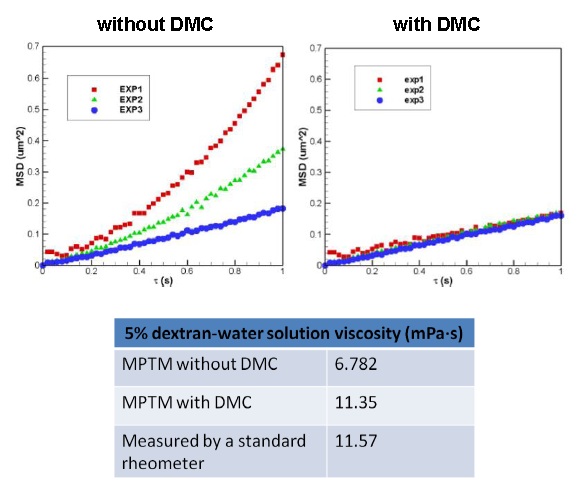 |
Figure 10. Giant intracranial aneurysm in the vertebrobasilar system of a diseased patient (LEFT) and the geometry of
the corresponding computational model (RIGHT).
 |
| In this project, my interest is to develop state-of-the-art methods for accurate rheological measurement of circulating cells (e.g., leukocytes,
platelets, tumor cells), blood and other biological fluids, and reactive materials which properties can change with time due to polymerization dynamics and other
active processes. Specifically, we are developing the following rheological methods: 1) acoustic tweezing rheometry,
2) multiple-particle-tracking microrheology for live cell measurements, 3) slotted rotor/slotted plate techniques for accurate characterization of blood rheology.
We also develop computational models for the flow of blood and other complex fluids, characterized by non-Newtonian viscoelastic properties, in channels of different
geometry (e.g., corresponding to the geometry of rheometric systems, capillary sprouts, and aneurysm sacs).
Multiple-particle-tracking microrheology (MPTM) is a noncontact method well suited for measurement of local rheological properties of
biological materials including circulating cells. Here, spherical particles of small size are suspended (or they can be naturally present) in a quiescent sample.
The trajectories of these particles are recorded by a high-speed camera attached to a microscope. Assuming the particles undergo random motion, the Stokes-Einstein
relationship is applied to the mean-square-displacement (MSD) vs. lag time data to determine the rheological properties of the sample. The biggest issue with MPTM is
the absence of the theory that adequately describes particles' trajectories. This leads to significant errors in rheological measurements with MPTM, especially when
studying living cells. The Stokes-Einstein theory works only if we neglect hydrodynamic interactions between particles. Another issue is that it is impossible to
completely eliminate the deterministic motion of a test fluid during measurement because of thermal convection of the fluid, fluctuations and inclination of
the experimental platform, and active transport of particles as, for example, in the case of cytoskeleton remodeling. Our laboratory works on the development of
the improved version of MPTM that implements the “deterministic motion cancellation” algorithm in analysis of the experimental datamicr. We have conducted
microrheological experiments with different fluids (water, dextran-in-water and agarose-in-water solutions) and showed that the deterministic motion cancellation
led to the values of the shear viscosity of these solutions that agreed much better with the published data than the values measured by conventional MPTM (Fig. 9).
Slotted rotor / slotted plate techniques. To reduce wall slip effects on rheological measurement, we work on a novel design of a rotational rheometer in which
the rotor has open slots. We also develop a slotted plate device for sensitive measure of blood yield stress and viscosity. In collaboration with Dr. De Kee
(Prof. of Chemical & Biomedical Engineering at Tulane University), we have tested these ideas via computational fluid dynamics (CFD) simulations. Our computational
work indicates that 1) a rheometer equipped with a slotted rotor can measure the fluid properties with enhanced accuracy and less sensitivity to the wall slip velocity
than a rheometer with a non-slotted rotor; 2) a double concentric cylinder rheometer with slotted rotor (DCCR/SR) is able to accurately measure rheological properties of
a wider spectrum of test fluids than a vane rheometer because of significant reduction of the end and secondary flow effects; and 3) wall slip effects and pressure drag
force can be substantially reduced by adopting a thin plate with sharp front and rear edges, high slot area ratio, and large number of slots, thus increasing sensitivity of
the slotted plate device to yield stress measurement. Using these data, we plan to develop prototypes of slotted plate and slotted rotor rheometers for accurate
measurement of rheological properties of human blood. To complete these tasks, we have established collaboration with TA Instruments, a leading manufacturer of
rheometric systems.
CFD modeling of blood flow. We have developod computational models of blood flow that predict the distribution of fluid shear stresses in capillaries with sprouts.
In these models, we use sprout geometries typical for microvasculature and considered non-permeable and permeable vessel wall conditions. We also have computational models that
simulate blood flow in the human vertebra-basilar system (ones of the major arteries in the brain) under normal conditions and in the presence of one or several aneurysms
(including the case of a giant aneurysm formed as a result of coalescence of two aneurysms, Fig. 10). The geometry of these models (Fig. 10) was reconstructed from
the images of the vessels extracted from deceased patients. Using the models, we have determined "weak spots" in the vessel walls at which new aneurysms can develop.
We also studied whether surgical treatment of intracranial aneurysms (e.g., filling an aneurysm with wires or Onyx solution) may lead to further complications.
Additionally, we showed that 1) yield stress fluids can be used for aneurysm embolization provided the yield stress value is 20 Pa or higher, 2) there exists a strong flow
recirculation in the empty aneurysm which is reduced by coil treatment but can be minimized by yield stress fluid treatment, and 3) the size of the inflow jet impingement
zone on the aneurysm wall is reduced most considerably when the aneurysm is filled with a yield stress fluid material.
|